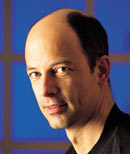
cached image
Markus Meister - US grants
Affiliations: | Molecular & Cellular Biology | California Institute of Technology, Pasadena, CA |
Area:
RetinaWebsite:
https://sites.google.com/site/markusmeisterlab/We are testing a new system for linking grants to scientists.
The funding information displayed below comes from the NIH Research Portfolio Online Reporting Tools and the NSF Award Database.The grant data on this page is limited to grants awarded in the United States and is thus partial. It can nonetheless be used to understand how funding patterns influence mentorship networks and vice-versa, which has deep implications on how research is done.
You can help! If you notice any innacuracies, please sign in and mark grants as correct or incorrect matches.
High-probability grants
According to our matching algorithm, Markus Meister is the likely recipient of the following grants.Years | Recipients | Code | Title / Keywords | Matching score |
---|---|---|---|---|
1993 | Meister, Markus | R01Activity Code Description: To support a discrete, specified, circumscribed project to be performed by the named investigator(s) in an area representing his or her specific interest and competencies. |
Multineuronal Signaling by the Vertebrate Retina @ Harvard University This research project aims for a detailed understanding of how the eye signals visual information to the brain. Functional investigations of the retina have primarily determined the properties of single neurons and their synaptic connections, while comparatively little is known about how a given cell's activity correlates in time with that of others. Yet the visual centers in the brain draw on the pattern of simultaneous firing in a large population of retinal ganglion cells in order to detect important features of our visual environment. Thus, the ultimate goal of the proposed work is to determine precisely how the retina encodes a visual scene by the patterns of electrical activity in the ganglion cell layer. The work focuses on the representation of visual information in a population of neurons, rather than individual cells. In these experiments, the isolated retina of a tiger salamander is placed on a glass surface with many embedded metal microelectrodes, covering an area of about 0.2mm(2). The retinal ganglion cells lie in close proximity to the electrode array, such that each site records extracellular action potentials generated by a few nearby neurons. Thus, one can monitor simultaneously the electrical signals from up to 100 ganglion cells. The retina is stimulated with sensory input by projecting an image from a computer monitor onto the photoreceptor layer. These methods will be used in the following specific projects: (1) A survey of the functional properties of ganglion cells in the retina. This study will determine the numerical proportions among cells of different response types, whether they are arranged in a spatially organized pattern, and how their distribution relates to the type of information they can extract from the visual stimulus. (2) A study of correlations between the firing patterns of different ganglion cells. These neurons are often strongly correlated in their activity, and participate in modes of concerted firing that can only be observed by multielectrode recording. Do these distributed firing patterns convey visual information? (3) An investigation of how features of the visual stimulus might be estimated from the recorded ganglion cell spike trains. The goal is to construct an algorithm that can interpret the retinal output to provide a reconstruction of the visual input. This will uncover the structure of the retinal code and suggest how circuits at subsequent visual centers might perform similar tasks of feature detection. These studies will reveal important aspects of collective function in the circuits of the retina. The results will provide a better understanding of how we see, and how the brain represents and processes information. Ultimately, this may lead to improvements in detecting and treating visual deficiencies, and, in the far future, to the development of visual prostheses that can emulate the function of the retina. |
0.903 |
1994 — 1995 | Meister, Markus | R01Activity Code Description: To support a discrete, specified, circumscribed project to be performed by the named investigator(s) in an area representing his or her specific interest and competencies. |
Multineuronal Signaling by the Retina @ Harvard University This research project aims for a detailed understanding of how the eye signals visual information to the brain. Functional investigations of the retina have primarily determined the properties of single neurons and their synaptic connections, while comparatively little is known about how a given cell's activity correlates in time with that of others. Yet the visual centers in the brain draw on the pattern of simultaneous firing in a large population of retinal ganglion cells in order to detect important features of our visual environment. Thus, the ultimate goal of the proposed work is to determine precisely how the retina encodes a visual scene by the patterns of electrical activity in the ganglion cell layer. The work focuses on the representation of visual information in a population of neurons, rather than individual cells. In these experiments, the isolated retina of a tiger salamander is placed on a glass surface with many embedded metal microelectrodes, covering an area of about 0.2mm(2). The retinal ganglion cells lie in close proximity to the electrode array, such that each site records extracellular action potentials generated by a few nearby neurons. Thus, one can monitor simultaneously the electrical signals from up to 100 ganglion cells. The retina is stimulated with sensory input by projecting an image from a computer monitor onto the photoreceptor layer. These methods will be used in the following specific projects: (1) A survey of the functional properties of ganglion cells in the retina. This study will determine the numerical proportions among cells of different response types, whether they are arranged in a spatially organized pattern, and how their distribution relates to the type of information they can extract from the visual stimulus. (2) A study of correlations between the firing patterns of different ganglion cells. These neurons are often strongly correlated in their activity, and participate in modes of concerted firing that can only be observed by multielectrode recording. Do these distributed firing patterns convey visual information? (3) An investigation of how features of the visual stimulus might be estimated from the recorded ganglion cell spike trains. The goal is to construct an algorithm that can interpret the retinal output to provide a reconstruction of the visual input. This will uncover the structure of the retinal code and suggest how circuits at subsequent visual centers might perform similar tasks of feature detection. These studies will reveal important aspects of collective function in the circuits of the retina. The results will provide a better understanding of how we see, and how the brain represents and processes information. Ultimately, this may lead to improvements in detecting and treating visual deficiencies, and, in the far future, to the development of visual prostheses that can emulate the function of the retina. |
0.903 |
1994 — 2003 | Meister, Markus | N/AActivity Code Description: No activity code was retrieved: click on the grant title for more information |
@ Harvard University This Presidential Faculty Fellow award funds research and teaching activity of a neuroscientist. Research concentrates on how large assemblies of neurons function, using as an the retina of the eye as a model system to study the complex interactions for collective signaling. Novel instrumentation is used to monitor the simultaneous activity of many nerve cells at once, to determine how a visual image is processed into a signal to be sent to the brain by neural pathways to visual centers. The role of correlated neural activity also appears to be important in development of a properly functional retina, and these studies explore how such mechanisms may be important in the adult retina. Teaching emphasizes to undergraduates and to graduates how the nervous system acts to integrate complex functions, with an outreach component into the local high schools. The impact of this project will be strong on visual neuroscience and computational neuroscience, and likely to other areas of brain information processing; the teaching component will be important to the scientific development of students over a wide range of ages and potential careers. |
0.903 |
1997 — 2000 | Meister, Markus | R01Activity Code Description: To support a discrete, specified, circumscribed project to be performed by the named investigator(s) in an area representing his or her specific interest and competencies. |
Neural Code of the Vertebrate Retina @ Harvard University All information for vision reaches the brain through action potentials coursing along the optic nerve fibers. This research project aims to determine precisely how the visual scene is encoded in these neural messages. This is of fundamental importance, both for understanding the function of the retina, which generates these signals, and for the interpretation of visual processes in the brain, which rely on these signals. Ultimately, these insights may lead to improvements in detecting and treating visual deficiencies, and, in the far future, to the development of visual prostheses that can emulate the function of the retina. Whereas previous functional investigations of the retina have primarily focused on the properties of single neurons and their synaptic connections, the present approach relies on monitoring the patterns of firing simultaneously in a large population of retinal ganglion cells. The isolated retina of a tiger salamander or a rabbit is placed on a glass surface with many embedded metal microelectrodes, covering an area of about 0.2 mm2, The retinal ganglion cells lie in close proximity to the electrodes, and thus one can monitor simultaneously the extracellular action potentials from 50 or more ganglion cells. The retina is stimulated with sensory input by projecting an image from a computer monitor onto the photoreceptor layer. Comparison of the retinal response with the visual stimulus will assess the neural code used by the retina, with the following specific aims: (1) To investigate the retinal circuits that underlie synchronous firing among groups of retinal ganglion cells. (2) To assess what mechanisms give rise to long-range synchrony in ganglion cell firing, and how these interactions shape visual processing. (3) To determine the nature of the neural code employed by the retina, specifically under what conditions it is stochastic or deterministic. (4) To study how the retinal code adapts to the properties of the visual environment, specifically whether it adjusts to scene statistics other than the mean intensity, such as the intensity contrast. |
0.903 |
1998 | Meister, Markus | N/AActivity Code Description: No activity code was retrieved: click on the grant title for more information |
Retinal Neurobiology and Visual Processing: 1998 Faseb Summer Research Conference: Saxtons River, Vt @ Federation of Amer Societies For Exper Biology The retina is the nerve tissue inside the eye, forming a complex processing stage for visual information. It is the crucial first stage where light reception starts to turn into vision. This conference is the fourth biennial one in a series devoted to the retina. The five day meeting will cover issues of retinal development, cellular functions that support neural signaling in the retina, neural circuits in the retina that process visual information, coding of information in the optic nerve from the retina to the brain, and some of the resulting abilities and limitations of visual behavior. The conference format is designed to promote a lot of discussions, not simply formal talks, and includes two workshops. This meeting will have an impact on visual neuroscience, and because of the emphasis on fostering the careers of young investigators, it will also have an important impact on the infrastructure of neuroscience. |
0.907 |
2003 — 2006 | Meister, Markus | R01Activity Code Description: To support a discrete, specified, circumscribed project to be performed by the named investigator(s) in an area representing his or her specific interest and competencies. |
The Neural Code of the Vertebrate Retina @ Harvard University [unreadable] DESCRIPTION (provided by applicant): This research aims at a detailed description of how the eye communicates with the brain. We want to understand the precise rules by which the action potentials of optic nerve fibers encode the visual stimulus presented to the eye, and how these rules are implemented by the neural circuitry of the retina. This will be achieved through a combination of methods: Multi-electrode array recording allows us to monitor in parallel the visual responses of many retinal ganglion cells in the isolated retinae of salamander and rabbit. Simultaneously, we will access individual interneurons with an intracellular microelectrode; recording and stimulation of that cell will reveal its role in visual processing pathways. Finally, mathematical models of retinal light responses will integrate these observations into an overall understanding of circuit function. The specific aims are: (1) to understand the circuit mechanisms that produce synchronous firing among retinal ganglion cells; (2) to investigate the effect of fixational eye movements on retinal processing, and the function of ganglion cells selective for differential motion; (3) to determine how the retina adapts to the statistics of the visual environment, and whether it performs a generalized form of novelty detection; (4) to construct mathematical models of retinal processing that can accurately predict ganglion cell firing from an arbitrary visual stimulus. Results from this work will lead to a better understanding of vision, and how the retina contributes to the overall process. They may also aid in future therapies, such as a retinal prosthesis that emulates the function of neural circuits in the retina. [unreadable] [unreadable] |
0.903 |
2003 — 2013 | Meister, Markus | R01Activity Code Description: To support a discrete, specified, circumscribed project to be performed by the named investigator(s) in an area representing his or her specific interest and competencies. |
Neural Computation From Retina to Visual Cortex @ California Institute of Technology Project Summary This research aims at understanding how visual information is processed in the eye and brain. Vision begins in the retina, a complex network of neurons in the back of the eye where visual images are converted into streams of action potentials that travel through the fibers of the optic nerve to the brain. There the signals pass through the thalamus to the visual cortex, where much larger circuits of neurons are brought to bear. Recent research has changed our view of the retina: Whereas it used to be considered a simple prefilter for the visual image, new results suggest that the retina computes quite specific features of the scene and conveys those to the brain through many parallel channels. The proposed research will build on this work, both to further our understanding of how the retina converts images into spike trains, and to investigate how this code for visual scenes can be used by the brain for further processing. This will be addressed in a collaborative project that combines experimental as well as theoretical and computational approaches. The specific aims are: (1) to unify diverse functions of the retina under a common mathematical formalism, and use this to discover new functions; (2) to determine how our brain might process retinal signals for rapid understanding of a new scene; (3) to explain how our fine vision can be so acute, given that the eyes are never holding still. If successful, this research will offer benefits on multiple fronts. First, it will expand our notions of what a neural circuit like the retina can compute and how the information is encoded in its output, an important goal of systems neuroscience. Second, it will help resolve two mysteries of visual processing, relating to both its remarkable speed and its high acuity. Third, progress in these areas will benefit brain science in general. Many of the circuit motifs encountered in the retina are repeated in other brain areas, and may well serve similar network-level functions. Finally, an improved understanding of early visual function can change how one thinks about visual diseases and therapy. For example, it now appears that certain functions previously assigned to the visual cortex already happen in the retina; if so, then retinal dysfunction could also have much more elaborate effects on visual experience. Conversely, in efforts to treat retinal degeneration by electronic or genetic prostheses one needs to know which aspects of retinal function the prosthesis should emulate to support visual perception. |
1 |
2009 — 2010 | Meister, Markus Sanes, Joshua R (co-PI) [⬀] |
RC1Activity Code Description: NIH Challenge Grants in Health and Science Research |
Transgenic Strategy to Map Structure and Function of Neural Circuits in Retina @ Harvard University DESCRIPTION (provided by applicant): This application addresses broad Challenge Area 06, Enabling technologies, specific Challenge Topic 06-NS- 106: "Validating new methods to study brain connectivity." The long-term goal of this research is a fundamental understanding of how the eye communicates with the brain. More immediately, the research serves to validate and improve a set of genetic tools for the study of neural circuits. The retina is a complex network of neurons in the back of the eye that converts a visual image into streams of action potentials that travel through the fibers of the optic nerve to the brain. The circuits of the retina begin with the photoreceptor cells that sense the light, pass through bipolar cells and other interneurons, and end with the ganglion cells that form the optic nerve. In all, the retina uses over 50 different types of neurons;the ganglion cells alone comprise about 20 different types. Each of these ganglion cell types extracts and reports a different aspect of the visual scene. A long-term goal of visual neuroscience is to understand what kind of visual processing occurs in each of these streams, and how those processes are implemented by the elaborate neural circuitry of the retina. New genetic methods are beginning to accelerate our understanding of neural systems. In particular, there has been great interest in finding genetic markers that distinguish the brain's many different neuron types. Experience has shown that availability of such a marker, in combination with new molecular and physiological approaches, can dramatically accelerate scientific progress on the structure and function of the corresponding neural circuits. Here we propose to apply these methods to assemble a complete catalog of the ganglion cell types in the mouse retina and to analyze their visual functions. The specific research goals are: (1) to find genes that are expressed specifically in one type of retinal ganglion cell;(2) to construct transgenic mice based on these genes in which all neurons of a given type are marked;(3) to exploit these lines for targeted studies of the structure and function of retinal pathways. For each type of retinal ganglion cell, we will determine the distribution of the neurons across the retina, how their dendritic fields cover visual space, and where in the brain their axons project. At the single-cell level we will examine the shape and location of the ganglion cell's dendritic tree within the retina to deduce its likely synaptic partners among retinal interneurons. To analyze visual function, we will determine what image features each ganglion cell type extracts from the visual scene. In addition, we will assess its involvement in ecologically important computations, such as the processing of image movement, and adaptation to the visual environment. This research will lead to a qualitatively new understanding of retinal function. It will inform our understanding of higher visual areas that draw all their input from the retina. Furthermore, the work will validate and gather experience with a set of genetic tools that can generalize to all brain circuits. PUBLIC HEALTH RELEVANCE: This project concerns basic research into the function of brain circuits. It will develop and test new genetic methods for visualizing types of nerve cells, and exploit these markers to understand how the circuits process information. In the long run, this will enhance our understanding of how the brain works, and how it fails in certain disorders. |
0.903 |
2011 — 2015 | Lichtman, Jeff W [⬀] Meister, Markus Sanes, Joshua R (co-PI) [⬀] Seung, Hyunjune Sebastian |
R01Activity Code Description: To support a discrete, specified, circumscribed project to be performed by the named investigator(s) in an area representing his or her specific interest and competencies. |
High Resolution Connectomics of Mammalian Neural Circuits @ Harvard University DESCRIPTION (provided by applicant): The overall goal of this work is to develop and validate a new suite of technologies that can rapidly and routinely generate circuit diagrams of nervous system tissue, sometimes called connectomes. The small size of neuronal processes and the synapses that connect them require that reconstruction be done at nanometer resolution;the distributed nature of neuronal connectivity requires reconstruction of large volumes, extending over a millimeter or more. Our method meets these two seemingly incompatible challenges by combining novel sectioning, electron microscopic imaging and reconstruction technologies. Together, these advances will allow us to acquire data and map circuits at least 1000-fold faster than has previously been possible. As a first test, we will reconstruct the retinal circuit of a mouse in its entirety. Enough is known about retinal structure and function to make this an appropriate tissue to validate the method. At the same time, this background will allow us to pose and solve important problems about neural circuits that will be directly applicable to the brain. We will then use the method to compare neural circuits in young adult and aged retina, providing insight into the structural basis of age-related neural decline. Finally, we will test the application of this connectomic method to human tissue. The new methods introduced here will transform neuroscience in several ways. First, it will allow elucidation of the structural underpinnings of brain function. It will also provide insight into how neural circuits are refined in early life and altered in old age. Second, applied to the ever increasing number of animal models of human behavioral disorders, it will help researchers delve into pathologies of cognition, behavior, and affect, some of which likely arise from miswiring of neural circuits. Finally, the method can be applied to any biological tissue where three-dimensional reconstruction of multiple large-volume specimens would be informative. PUBLIC HEALTH RELEVANCE: The connectome of each individual is probably unique, containing the traces of experiences and learned behaviors. Until we can acquire and decipher connectomic patterns, we will not know how acquired information is organized and encoded in the brain, or whether, as many suspect, miswiring underlies a variety of developmental, aging, and behavioral disorders. As the human genome project demonstrated, biomedical science can be transformed by scaling up and speeding up technologies to the point where they can provide comprehensive information about essential biology;our brain circuit reconstruction will be fast enough to compare entire circuits from multiple individuals at nanometer resolution. |
0.903 |
2019 — 2021 | Meister, Markus | R01Activity Code Description: To support a discrete, specified, circumscribed project to be performed by the named investigator(s) in an area representing his or her specific interest and competencies. |
Neural Computation For Innate Behaviors in the Superior Colliculus @ California Institute of Technology Neural computation for innate behaviors in the superior colliculus The long-term goal of the proposed research is to understand how the brain makes sense of the onslaught of sensory data to extract just the few bits of relevant knowledge needed to make a decision. Specifically we will focus on innate behaviors of the laboratory mouse, such as the escape from a threat, the pursuit of small prey, and visual navigation. These are robust and reliable behaviors that require rapid and sensitive detection and localization of specific features in the visual scene. Mammals have two brain areas dedicated to visual processing: the thalamo-cortical pathway and the superior colliculus. The superior colliculus (SC) is the evolutionarily more ancient pathway, shared with non-mammalian vertebrates. It receives direct sensory input from the retina, and its output neurons produce motor signals that can steer the animal's movements. In general terms, the superior colliculus is thought to identify the most salient points in the scene for the purpose of overt orienting actions. It may also direct the orienting of covert neural resources, like when we ?pay attention? to a location in the scene. In recent research we identified three aspects of neural computation in the SC that extend much beyond the processing performed in the retina. On the background of this work, combined with earlier analysis, we developed a hypothetical model of neural processing within the SC. Here we propose to test key aspects of this model, with the goal of developing its quantitative details such that it correctly accounts for the extraction of salient image features from the visual scene. The research combines behavioral methods, large-scale electrical and optical recordings of neural activity, manipulations of neural circuitry, circuit tracing tools, and circuit modeling. We will pursue the following aims: (1) Understand the unusual functional anatomy of the SC whereby different parts of the visual field emphasize different visual features. (2) Determine how the SC achieves the extraction of select visual features with both specificity and invariance. (3) Understand an intriguing form of image memory in the SC that suppresses the response to familiar stimuli. Two common themes connect these aims: Do these neural computations require a contribution from the visual cortex? And what is the role of different cell types in the underlying neural circuits? If these aims are achieved, they will contribute to an integrated understanding of how the brain reliably and automatically identifies the most salient features of the environment, an essential function for our interactions with the world. In turn that will help illuminate failure of that process, as occurs in various psychiatric disorders of attention. |
1 |
2019 — 2021 | Meister, Markus | T32Activity Code Description: To enable institutions to make National Research Service Awards to individuals selected by them for predoctoral and postdoctoral research training in specified shortage areas. |
Predoctoral Training in Quantitative Neuroscience @ California Institute of Technology 7. Project Summary/Abstract This proposal will create a program for Predoctoral Training in Quantitative Neurosciences at Caltech. The field of neuroscience is currently experiencing explosive growth, driven by a plethora of new technologies for observing and manipulating the brain. To apply these methods fruitfully and interpret the resulting flood of data, modern neuroscientists need a sophistication in mathematical and computational approaches that traditional neuroscience training programs cannot provide. At the same time we are seeing a convergence of understanding at different levels of brain organization, ranging from the molecules that specify neural connections to the dynamic function of large circuits in the human brain. Thus, there is an urgent need to train young neuroscientists with an integrated perspective that spans many levels ? from molecules to behavior to computation ? which have traditionally been taught in separate programs. Caltech is in an ideal position to answer these demands. It is an institution almost entirely dedicated to scientific research, routinely ranked among the top few universities in the world. Caltech is well known for its rigorous training in physics, mathematics, and engineering, but it also has a distinguished history in biological research, and in the neurosciences in particular. The small size supports a climate in which scientific collaboration across disciplines is effortless. The present proposal exploits these institutional strengths to create a new environment for training in quantitative neuroscience. The program is focused on PhD students in years 1 and 2. The strategy begins with highly selective recruiting of candidates from a broad range of undergraduate majors, ranging from biochemistry to psychology to computer science. First-year students get introduced to faculty research during three rotations of 3 months each, after which they choose a primary mentor to supervise their PhD research. Trainees also complete a rigorous 2-year course curriculum that ensures core competency in the following six areas: (i) molecular and cellular neuroscience; (ii) systems and computational neuroscience; (iii) human neuroscience and brain disorders; (iv) tools and technology for neuroscience; (v) applied mathematics and statistics; (vi) scientific programming and data analysis. Trainees get writing experience through a mentored process of fellowship applications. They also give numerous oral presentations in forums on and off campus. After a candidacy examination at the end of year 2, trainees focus on PhD research, with the aim of authoring one or more major publications, and graduating by year 6 or earlier. Each trainee's trajectory through the program is accompanied by individualized advising, adjusting coursework to ensure broad competency while also challenging the student in a domain of special expertise. In summary, the proposed program in quantitative neurosciences responds in a timely manner to an urgent demand for a new type of graduate training in this rapidly evolving discipline. |
1 |
2020 | Meister, Markus Wagenaar, Daniel A (co-PI) [⬀] |
R21Activity Code Description: To encourage the development of new research activities in categorical program areas. (Support generally is restricted in level of support and in time.) |
Towards Non-Invasive Magnetic Control of Cells - a Global Search For Magnetoreceptors @ California Institute of Technology ABSTRACT Towards noninvasive magnetic control of cells ? a global search for magnetoreceptors If biological cells could be controlled using magnetic fields, this would enable unprecedented approaches to basic biology and medicine. Ideally one would want a genetic tool that can render arbitrary cells magnetically sensitive, a goal now known as ?magnetogenetics?. Several attempts to accomplish this by de novo bioengineering have failed, mainly because magnetic fields interact only weakly with biological molecules. At the same time we know that certain animal species have the remarkable ability to sense the Earth's magnetic field and to use this information for orientation and navigation. Thus there must exist nerve cells with the mechanism to transduce even weak magnetic fields: the magnetoreceptors. If one could find those receptor neurons, they would reveal a cellular pathway that could be used for magnetic control. Remarkably the identity and mechanism of magnetoreceptor neurons is still unknown. The approach proposed here is to first search for neural signals anywhere in the brain that respond to magnetic stimuli. Based on those signals one can engage a magnetic scanning method to localize where the magnetic responses originate. Ultimately this will lead to the receptor cells. The first and essential step is to find an unambiguous neuronal response to magnetic fields in any species. The research presented here will accomplish this through a collective science project that will coordinate many laboratories for a short duration. These days scores of research groups are engaged in high-throughput neuroscience pursuing a broad range of questions in diverse species. Revolutionary improvements in the tools of neurophysiology enable experiments that routinely record signals from hundreds to thousands of neurons at a time. The project will transiently engage about 50 of these laboratories in a broad unbiased search for magnetoreceptors. Building on personal contacts the PI has already secured agreement from an illustrious list of pilot collaborators to offer experimental time and share data. The Caltech team will construct electromagnetic stimulators that produce a defined magnetic field and ship these to each partner lab. The device can be added easily to an ongoing experiment, and a mere 20 minutes of recording will reveal whether any of the neurons under study carry magnetic signals. The team will collect all the resulting data and analyze them for magnetic responses. A positive finding will immediately be subject to independent replication. By the end of the two-year period the project is expected to screen several million neurons in many different animal species and brain areas for magnetic responses, at least a 100-fold increase over the cumulative effort to date. If this exploratory research program yields magnetoreceptors in any species, that will set the stage for future work that unlocks their biophysical mechanisms and ultimately realizes the dream of magnetogenetics. |
1 |