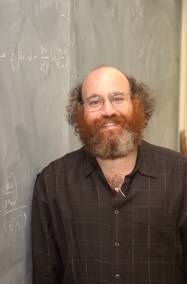
William Bialek - US grants
Affiliations: | 1998-2002 | Marine Biological Laboratory, Falmouth, MA, United States | |
2001- | Princeton University, Princeton, NJ |
Area:
Computation & TheoryWebsite:
https://www.princeton.edu/physics/people/display_person.xml?netid=wbialek&display=facultyWe are testing a new system for linking grants to scientists.
The funding information displayed below comes from the NIH Research Portfolio Online Reporting Tools and the NSF Award Database.The grant data on this page is limited to grants awarded in the United States and is thus partial. It can nonetheless be used to understand how funding patterns influence mentorship networks and vice-versa, which has deep implications on how research is done.
You can help! If you notice any innacuracies, please sign in and mark grants as correct or incorrect matches.
High-probability grants
According to our matching algorithm, William Bialek is the likely recipient of the following grants.Years | Recipients | Code | Title / Keywords | Matching score |
---|---|---|---|---|
1987 — 1991 | Bialek, William | N/AActivity Code Description: No activity code was retrieved: click on the grant title for more information |
Presidential Young Investigator @ University of California-Berkeley This Presidential Young Investigator award is for Dr. William N. Bialek, an Assistant Professor of Biophysics and Physics. His research interests are in the application of theoretical approaches to biological problems, especially problems in sensory physiology and biophysics. Examples of the areas in which he has contributed include: 1. photochemical processes in photosynthesis, 2. the roles of protein structure and dynamics in oxygen transport by myoglobin solutions, 3. the mechanism by which hearing thresholds can be below the estimates of thermal noise levels, 4. the mechanism by which photon absorption can lead to visual nerve impulses, 5. the relation of neural impulse frequency to information coding. The PI intends to continue to explore these and related areas of biophysics, applying theoretical concepts to a number of problems. This approach is greatly underutilized, probably because the overhwelming majority of biological scientists do not have the extensive background in physics nor do most physicist have sufficient awareness of the biological problems that would profit from these methods. In addition, the PI appears to be a most remarkable young investigator, with depth of understanding in physics and what one evaluator describes as "stunning breadth of knowledge". There is no doubt that our understanding of various sensory processes can benefit tremendously from the efforts of Dr. Bialek. This amply demonstrated by the importance of his contributions during the few years that he has been active. |
0.951 |
2000 — 2002 | Bialek, William | R25Activity Code Description: For support to develop and/or implement a program as it relates to a category in one or more of the areas of education, information, training, technical assistance, coordination, or evaluation. |
Training in Methods of Computational Neuroscience @ Marine Biological Laboratory The goal of the Methods in Computational Neuroscience Course at the Marine Biological Laboratory is to train 22 advanced students and post-doctoral fellows a year in the fundamental intellectual issues in computational neuroscience and to avail them with analytical and numerical tools to assist in their studies. The course has unique features that would be hard if not impossible to replicate in a university setting. First, it continues to attract and educate students from a broad range of established disciplines that impact upon neuroscience, including biology, cognitive science, computer science, engineering, mathematics and physics. Secondly, as a means to provide a coherent and relatively complete perspective on the growing field of computational neuroscience, students are exposed to a large visiting faculty in addition to receiving instruction and guidance from directors, scholars-in-residence and course assistants. It is unlikely that such a combination of faculty could be convened for an analogous course in a university setting. Lastly, the course provides students with access to state-of-the-art computational techniques and computer hardware fundamental to simulating neural systems from the detailed cellular through more abstracts systems level. The course is organized as an intensive four week lecture and laboratory series. The lectures progress along an increasing scale of computational complexity. They initially focus on the electrophysiology and chemical dynamics of individual neurons and synapses. This is followed by lectures on the dynamics and plasticity of local neuronal circuits and small nervous systems, topics that illustrate how single-cell properties shape computations by small networks. A final series of lectures addresses computations that involve sensory and perceptual issues in large neuronal systems, particularly cortex, and the emerging application of abstract models to provide a framework for understand these systems. The laboratory emphasizes the spectrum of tools necessary for successful research in computational neuroscience. On the one hand, the course provides training with general purpose simulation software as well as with simulation software that is specific to single cell dynamics, or to both single cells and large networks. On the other hand, students are trained in areas of applied mathematics, such as probability theory and matrix algebra, that are essential to formulating and solving problems in the field. |
1 |
2004 — 2008 | De Ruyter Van Steveninck, Rob [⬀] Bialek, William |
N/AActivity Code Description: No activity code was retrieved: click on the grant title for more information |
Optimal Computation of Flow Field Variables From Natural Visual Signals @ Indiana University Optimal computation of flow field variables from natural visual signals. |
0.961 |
2006 — 2009 | Bialek, William | R01Activity Code Description: To support a discrete, specified, circumscribed project to be performed by the named investigator(s) in an area representing his or her specific interest and competencies. |
Dynamics, Scaling, and Precision of Morphogen Gradients in the Drosophila Embryo @ Princeton University The overall goal of our research effort is to produce a quantitative description, and ultimately a mathematical theory, of how spatial patterns of gene expression are established in a developing embryo. Our experimental system is the Bicoid (Bed)morphogen gradient in the Drosophila embryo, the primary maternal determinant of the anterior-posterior axis. Our project brings together modern methods of experimental and theoretical biophysics with those of molecular biology and genetics to provide an integrated attack on (1) how the Bed gradient is established and maintained, (2) how is it scaled proportionately across embryos of different size, and (3) how is it read out to produce precise patterns of downstream gene expression. The dynamics of the formation and stabilization of the Bed gradient will be measured in living embryos expressing eGFP-Bcd. Image sequences from time-lapse two photon microscopy will be used, together with photobleaching methods and computational analysis, to assess passive and active contributions to gradient dynamics, to determine the protein half life of Bed, and to determine absolute concentrations of Bed in nuclei and cytoplasm at various stages of development. The scaling of Bed and gap gene expression patterns across closely related dipteran species that have bodies of different size but almost identical proportions will be analyzed using classical staining methods, extended by more sophisticated image processing methods. In addition, transformants expressing eGFP labeled-bicoid genes from different sized fly species will be expressed in Drosophla melanogaster to probe the biophysical mechanisms behind this scaling. Although genes appear to be activated by Bed at specific concentration thresholds along the length of the embryo, noise in transcriptional regulation places limits on the accuracy with which such thresholds can be marked. Theoretical work will define the nature of these limits in progressively more realistic models of each regulatory step. To test these models, the mean and variance of target genes (hunchback, orthodenticle) will be measured as functions of local concentration of Bed,both in wild type embryos, and in mutants and genetic mosaics where levels and activities can be artificially manipulated. Spatial correlations in the variance will also be measured, testing the hypothesis that communication among nuclei plays a role in suppressing noise and enhancing the precision of developmental boundaries. Our project addresses the fundamental question of how small changes in the concentration of signaling molecules produce robust control of cell fate. Precise read-out of such signaling pathways is required for normal development. Perturbations in signaling are associated with birth defects and cancer in adults. |
1 |
2006 — 2011 | Bialek, William Berry, Michael (co-PI) [⬀] |
N/AActivity Code Description: No activity code was retrieved: click on the grant title for more information |
Combinatorial and Collective Coding in the Retina @ Princeton University Everything that we know about the visual world is built from the sequences of discrete electrical pulses that stream from eye to brain along the roughly one million individual cables that form the optic nerve. Until recently, when scientists wanted to "listen in" on these signals, all they could do was to monitor one cable or cell at a time, getting only a very limited view of the messages being passed through the visual system. In particular, this left us literally and figuratively blind to the possibility that combinations of pulses from neighboring cells could have special meaning. The emergence of experimental techniques for recording the signals from many cells simultaneously, under reasonably natural stimulus conditions, thus offers an unprecedented opportunity to explore the full complexity of the raw data that the brain has to work with as it tries to understand what we see. Indeed, the most recent generation of multi-electrode array technologies achieves a sampling efficiency that should allow recording from nearly all of the cells in a small patch of the retina, providing access to all of the signals that the brain can use in making sense out of the corresponding small patch of the visual world. The goals of this project are to develop in tandem the experimental methods to realize this promise and the theoretical framework for understanding the problem that the brain has to solve in dealing with these data. |
1 |
2007 — 2019 | Machta, Benjamin (co-PI) [⬀] Callan, Curtis [⬀] Bialek, William Wingreen, Ned (co-PI) [⬀] |
N/AActivity Code Description: No activity code was retrieved: click on the grant title for more information |
Theoretical Physics of Biological Systems @ Princeton University Theoretical physics is the search for concise mathematical models of Nature. It has had great success in dealing with the inanimate world: we can now predict in quantitative detail what the most sensitive experiments will observe inside the nucleus and in the cosmos at large. By contrast, even as our ability to observe and measure improves dramatically, the phenomena of life remain largely unpredictable, even in their most qualitative aspects. In this project, a group of theoretical physicists will engage with students and postdoctoral scholars in an effort to close this gap; in short, to construct a theoretical physics of biological systems. The proponents have explored phenomena that span the tree of life: from metabolism in bacteria, through the determination of cell fate in embryonic development, to coding and computation of sensory information in brain. They have identified broad theoretical problems which cut across the traditional biological divisions of organism and system: Do living organisms operate near the limits set by the laws of physics as they gather and process information? Can we learn the detailed microscopic "model" of an organism, its "wiring diagram", from the finite set of observations we can make on how it behaves? How do organisms set the parameters that govern their function (i.e. how do they learn from experience)? These questions can all be given a mathematical form which guides a search for answers in terms of general principles, in the tradition of physics that will apply across disparate biological domains. The time is right to bring the beautiful phenomena of life under the powerful predictive umbrella of theoretical physics. Just as cosmology has progressed, in roughly one generation, from wild speculation to a precise framework for analyzing a rapidly expanding set of observations, the proponents believe that the intimate interaction between theory and experiment can lead to a new and deeper physics of biological systems. It is the creation of this scientific culture, where theory and experiment are equal partners in the exploration of life that is the fundamental intellectual merit of the project. It is not just the boundaries of academic disciplines, but our view of ourselves, which is at stake. A very important aspect of this project will be the training of a new generation of physicists for whom the development of a theoretical understanding of biological systems is a central part of their discipline. The graduate students and postdoctoral scholars who pass through the group will learn by example how to pursue that goal in a way consistent with the intellectual rigor and traditions of physics. They will eventually move on to faculty positions of their own, where they will transmit this attitude to new generations of students. More broadly, all project personnel are deeply engaged with new educational initiatives, addressing levels from the first year of college to advanced PhD students, which provide a more complete guide to the evolving, multidisciplinary intellectual landscape. |
1 |
2010 — 2014 | Callan, Curtis [⬀] Bialek, William Wingreen, Ned (co-PI) [⬀] |
N/AActivity Code Description: No activity code was retrieved: click on the grant title for more information |
The Theoretical Physics of Biological Systems @ Princeton University In this project the PIs will explore phenomena that span the tree of life: from metabolism in bacteria, through the determination of cell fate in embryonic development, to coding and computation of sensory information in the brain. They have identified broad theoretical problems which cut across the traditional biological divisions of organism and system: Do living organisms operate near the limits set by the laws of physics as they gather and process information? Can the detailed microscopic model of an organism, its wiring diagram be understood from the finite set of observations that can be made on how it behaves? How do organisms set the parameters that govern their function (i.e. how do they learn from experience)? These questions will be given a mathematical form, which will guide a search for answers in terms of general principles, in the tradition of physics, that will apply across disparate biological domains. The participants in the project will assemble into subgroups to attack instances of these problems. The individual projects will have unusual scope: as an example, the question whether the complex statistics of biological behavior can be captured in a learnable mathematical model will be asked in very similar terms both of spiking retinal neurons, and of the antibody sequence repertoire of individual zebrafish. These questions will be answered in the light of accurate data and the work will involve a close partnership with many experimental groups in fields ranging from bacteriology to human perceptual psychology. The product of these interactions will be the design of novel experiments and the creation of novel data analysis methods in order to address clearly formulated mathematical questions of broad significance. An important component of this project is the training of a new generation of physicists for whom the development of a theoretical understanding of biological systems is a central part of their discipline. The graduate students and postdoctoral scholars who pass through the group will learn by example how to pursue that goal in a way consistent with the intellectual rigor and traditions of physics. |
1 |
2010 — 2020 | Callan, Curtis (co-PI) [⬀] Bialek, William Shaevitz, Joshua [⬀] |
N/AActivity Code Description: No activity code was retrieved: click on the grant title for more information |
Collaborative Research: Pols Student Research Network @ Princeton University This collaborative research project, consisting of four institutions (Rice, Yale, UIUC and Princeton) aims to continue the Physics of Living Systems Student Research Network (PoLS SRN). This network has been in existence for four years and has had a dramatic impact on many graduate students, both in the US and abroad, working on the application of physical science techniques to living systems. These students now can participate in a global community that can help deal with the many complex issues involved in conducting research in such a new and inherently multidisciplinary field. These issues range from proper training, to gaining a broad perspective, to accessing technical expertise that may not be available at their home institution. In addition to the obvious broader impacts related to training of a research workforce, there are other broad impacts of this plan. Via the interaction of one of the PoLS nodes (Rice) with the biomedical community in Houston, students and faculty will be exposed to possible avenues whereby physics can contribute to human health issues. Funds to attract students from under-represented groups to network meetings will be available through the new funds administered by the newly proposed network coordinator. Also deas vetted by the PoLS SRN will be adapted to create student networks in other areas of science and engineering. |
1 |
2011 — 2014 | Bialek, William (co-PI) Shaevitz, Joshua W [⬀] |
R01Activity Code Description: To support a discrete, specified, circumscribed project to be performed by the named investigator(s) in an area representing his or her specific interest and competencies. |
A New Paradigm For Quantifying Animal Behavior in a Model Genetic System @ Princeton University DESCRIPTION (provided by applicant): Progress toward understanding and curing many human neurological diseases is hindered by a lack of methods for objective and quantitative assessment of the connections between genes and behavior. Traditional studies of animal behavior probe only a small subset of user-defined behaviors in low- dimensional data and are inappropriate for the discovery of subtle phenotypes or complex relationships between genes and behavior. To move beyond these simple representations of animal behavior, we propose to build a high-resolution, three-dimensional imaging system that can track single behaving animals. This system will quantitatively connect behavior to genetics using a data-driven method for the discovery of stereotyped motions. Our specific aims are to 1) develop a new standard for behavioral observation and automatic phenotyping to quantify the behavior of freely moving fruit flies, 2) demonstrate the sensitivity and accuracy of our new technology by studying Drosophila models of neurodegenerative diseases and the behavior of closely-related species of Drosophila fruit flies and 3) expand our system to enable simultaneous tracking and analysis of two independent flies in a single arena and test this system with assays of courtship behavior between male and female flies. The ability to measure subtle changes in behavior in a model genetic organism will bring us closer than we have ever been to understanding neurological diseases and behavioral disorders at the genetic and molecular level. |
1 |
2014 — 2015 | Kopell, Nancy (co-PI) [⬀] Bialek, William Bassett, Danielle [⬀] |
N/AActivity Code Description: No activity code was retrieved: click on the grant title for more information |
Workshop: Quantitative Theories of Learning, Memory, and Prediction @ University of Pennsylvania The development of quantitative theories of learning, memory, and prediction is fundamental to understanding human cognitive processing. This workshop, to take place in Arlington VA, May 8-9, 2014, tackles a key scientific need: to integrate modern complex systems and network approaches with understanding cognitive function. Predictive models of higher order cognitive processes could inform the development of neuroprosthetics, facilitate advances in brain-computer interfaces, and assist in the construction of intervention protocols for cognitive deficits that accompany neurological disorders and psychiatric disease. |
0.951 |
2014 — 2016 | Shaevitz, Joshua (co-PI) [⬀] Bialek, William Murthy, Mala [⬀] |
N/AActivity Code Description: No activity code was retrieved: click on the grant title for more information |
@ Princeton University Animals, from insects to humans, are inherently social, and brains have evolved to be most sensitive to sensory cues that carry social information (for example, speech sounds or pheromones). Very little is known regarding how animal brains process information in the context of social interactions. This proposal seeks to address this complex issue by focusing on the relatively simple nervous system of the fruit fly Drosophila, and takes advantage of the wealth of tools available in this system to dissect the mechanisms underlying social behaviors. The three principal investigators (Murthy, Shaevitz, and Bialek) are experts with behavioral analysis, theory/modeling, and neural circuit analysis, and will use several new methods to study courtship, a robust social behavior that has been shown to involve a complex interaction of a male and a female. This work will not only uncover the mechanism by which sensory inputs and internal states interact to generate behavior, but also benefit studies of disorders (e.g., autism spectrum) that impact the social brain. The research project is complemented by outreach efforts targeted at educating undergraduates, and in particular young women, in modern methods in computational neuroscience. |
1 |
2014 — 2016 | Bialek, William | N/AActivity Code Description: No activity code was retrieved: click on the grant title for more information |
Brain Eager: Toward a Theory of Real Neural Networks @ Princeton University Project: Our perception of the world seems a coherent whole, yet it is built out of the activities of many thousands or even millions of neurons, and similarly for our memories, thoughts, and actions. It seems difficult to understand the emergence of behavioral and phenomenal coherence unless the underlying neural activity also is coherent. But if the neurons are not independent, how do we describe their collective activity? In this work, The PI takes several approaches to this problem. In each case the PI is reaching for a theoretical framework with a generality that transcends the details of particular systems, but in each case the work is grounded by intimate collaboration with several experimental groups. It is now conventional to assert that the emergence of new, larger data sets in the study of biological systems creates a need for new and more efficient methods of data management and analysis. Thus, it is widely expected that the BRAIN initiative, which will provide substantial resources to advance our ability to record simultaneously from many neurons, will also have a significant "computational" component related to the storage, handling, and analysis of the huge bodies of data that will be generated. It is much less widely appreciated that making sense of the collective behavior in systems with many interacting degrees of freedom requires theory, not just analysis. The goal of this work is to develop such a theoretical framework. |
1 |
2014 — 2016 | Bialek, William (co-PI) Brody, Carlos D [⬀] Seung, Hyunjune Sebastian Tank, David W (co-PI) [⬀] Wang, Samuel Sheng-Hung (co-PI) [⬀] Witten, Ilana (co-PI) [⬀] |
U01Activity Code Description: To support a discrete, specified, circumscribed project to be performed by the named investigator(s) in an area representing his or her specific interest and competencies. |
Mechanisms of Neural Circuit Dynamics in Working Memory @ Princeton University ? DESCRIPTION (provided by applicant): Working memory, the ability to temporarily hold multiple pieces of information for mental manipulation, is central to virtually all cognitive abiliies. Working memory has been closely associated with multiple kinds of neural activity dynamics, such as persistent neural activity, activity ramps, and activity sequences. The neural circuit mechanisms of these dynamics remain unclear. This proposal will apply advanced technologies such as virtual reality, automated monitoring of behavior, in vivo microscopy, ontogenetic, and neural circuit reconstruction to solve fundamental problems in the understanding of working memory. The accumulation of evidence over time scales of seconds, a type of working memory critical for decision-making, will be used as a test bed for studying working memory. The proposal will build upon a rodent evidence-accumulation paradigm that allows quantitative, temporally precise parameterization of working memory and decision-making. The paradigm will be implemented with head-fixed rodents behaving in a virtual reality system (Aim 1), providing mechanical stability that enables the use of two-photon calcium imaging to observe neural activity related to working memory in the neocortex, basal ganglia, and cerebellum (Aim 3). Brain activity will also be perturbed using ontogenetic to probe the roles of brain regions and specific cell types in the formation and stabilization of memory (Aim 2). Finally, we will develop methods for probing the roles of cell types and connectivity in working memory through correlative serial electron microscopy and light microscopy as well as imaging of population responses to ontogenetic stimulation of single cells or groups of cells (Aim 4). This three-year project will produce a catalog of the types of neural circuit dynamics that are related to working memory across many brain regions. In subsequent years, this catalog will be mechanistically investigated by the anatomical and physiological methods developed in Aim 4. The long-term goal of this project is to arrive at a complete, brain-wide understanding of the cellular and circut mechanisms of activity dynamics related to working memory. The understanding is expected to take the form of a new generation of models containing cognitive variables distributed across brain regions, as well as models that explicitly represent neural circuit dynamics. This achievement will be a crucial step towards a mechanistic understanding of the neural basis of cognition. |
1 |
2017 — 2022 | Shaevitz, Joshua (co-PI) [⬀] Bialek, William |
N/AActivity Code Description: No activity code was retrieved: click on the grant title for more information |
Pfc: Center For the Physics of Biological Function @ Princeton University A physical and quantitative understanding of the processes of life and living systems must describe biological functions in different contexts and across a wide range of systems. Through this Physics Frontiers Centers award the Center for the Physics of Biological Function (CPBF) will probe the unifying physical principles that govern the emergence of essential biological functions. A joint effort of Princeton University and the Graduate Center of the City University of New York, the CPBF will bring together theorists and experimentalists to study biological functions in a quantitative and systematic manner across a range of diverse systems. From cells to organisms to groups of organisms the CPBF will explore functional behavior, the mechanics of biological networks, the impacts of limited physical information within systems, and how biological systems set efficient parameters. The CPBF will foster the training and development of a new and diverse generation of scientists at the interface of physics and biology. It will expand successful existing interdisciplinary programs for undergraduates and graduate courses, and develop a dedicated undergraduate summer school. In addition, the center will host seminars and symposia that will connect CPBF with the larger scientific community and will engage in public lectures in New York City. |
1 |
2018 — 2021 | Bialek, William Murthy, Mala [⬀] Pillow, Jonathan William (co-PI) [⬀] Shaevitz, Joshua W (co-PI) [⬀] |
R01Activity Code Description: To support a discrete, specified, circumscribed project to be performed by the named investigator(s) in an area representing his or her specific interest and competencies. |
Dissecting Sensorimotor Pathways Underlying Social Interactions: Models, Circuits, and Behavior @ Princeton University Project Summary/Abstract Social interactions across the animal kingdom, from courtship rituals and aggressive interactions to spoken conversation, are wondrously complex - they necessarily involve back- and-forth feedback between nervous systems transmitted through multiple sensory modalities and each animal's behavior. Typical experiments in this field observe only a tiny fraction of the activity in any neuronal circuit, and then only under a very limited range of behavioral conditions. To overcome this limitation, the proposed research leverages the compact nervous system of Drosophila melanogaster, combined with its wealth of genetic tools, to study the dynamic behavioral interactions and detailed neural mechanisms that underlie courtship between males and females. The project combines unbiased measurement of behavior, neural circuit manipulations, neural recordings in behaving animals, and sophisticated computational models. The specific aims include: i) elucidating the computations that the brain performs during courtship by mapping the sensorimotor transformations underlying male and female interactions over time via quantitative behavioral assays and the generation of predictive models; ii) combining models with neural perturbations to map the underlying circuits that govern the link between sensory inputs and behaviors; And, iii) testing the models of neural control during courtship by monitoring neural activity in behaving animals experiencing fictive courtship stimuli in a virtual-reality apparatus. This work will substantially advance our understanding of how two interacting brains process and transfer information, and will uncover general principles of neural circuit function that will inform studies of sensorimotor integration in more complex animals, such as rodents and humans. The project will also produce new experimental and theoretical tools for studying social behaviors. Finally, it will shed light on the mechanisms that go awry in several disorders, including Autism Spectrum Disorder (ASD), in which sensory perception becomes disentangled from motor outputs ? these disorders have profound effects on cognitive well-being and a major impact on public health. |
1 |
2020 | Bialek, William Palmer, Stephanie E [⬀] Schwab, David Jason (co-PI) [⬀] |
R01Activity Code Description: To support a discrete, specified, circumscribed project to be performed by the named investigator(s) in an area representing his or her specific interest and competencies. |
Coarse-Graining Approaches to Networks, Learning, and Behavior @ University of Chicago Project Summary The theory hub put forward in this proposal will work to translate successful and powerful approaches to describing emergent collective behavior in physical systems so they can be applied to the brain. Working closely together, the three theorists will develop methods for finding and quantifying the relevant modes of population activity in the brain, both in instantaneous snapshots of activity and activity as it evolves in time. Methods will be tested in a wide range of neural systems at different processing stages and scales: from salamanders to rodents to humans, from the retina to the cortex, from tens to thousands of cells. The approach will be validated by checking that the neural code can be read out with high fidelity even after being compressed into a much smaller subspace. The project will produce data analysis code that will be made available for neuroscience researchers to use on their own data, in addition to the results of the analyses of the particular systems studied. The neural code is inherently collective; while single neurons execute sophisticated computations, hundreds to thousands of neurons are utilized to sense the environment and drive behavior in even the simplest organisms. Although the past hundred years have yielded substantial progress in neuroscience, only recently have researchers had the capacity to record from complete neural populations - that is, to view the collective behavior of a functioning neural network. With these rapid experimental advances, there is an urgent need for complementary theoretical and computational approaches to guide the exploration of emergent behavior in large groups of neurons, allowing one to turn `big data' into `big ideas'. This proposal outlines a path towards a new theoretical framework for finding and quantitatively analyzing collective phenomena in the brain that underlie sensory coding, the representation of space, prediction, and ultimately drive behavior. The project draws heavily on the success of so-called renormalization group approaches in theoretical physics that revolutionized the understanding of collective phenomena in physical systems, and sculpted much of the progress in statistical physics in the second half of the twentieth century. The methods explored in this proposal generalize such techniques so they can be applied to a much wider range of problems. The methods developed by this theory hub based on the renormalization group will be applicable to a wide range of neural data since they are explicitly designed to generalize techniques from theoretical physics to a much broader setting. Indeed, a larger goal of the approach is to search for universality in collective behavior in the neural code. The techniques proposed are relatively straightforward to execute and will provide a fundamental methodology for interrogating high-dimensional data in fields as diverse as behavioral neuroscience and biophysics. The new techniques will also be taught as part of the three theorists' ongoing efforts to expose incoming graduate students in biological sciences to quantitative methods in biology. |
0.952 |